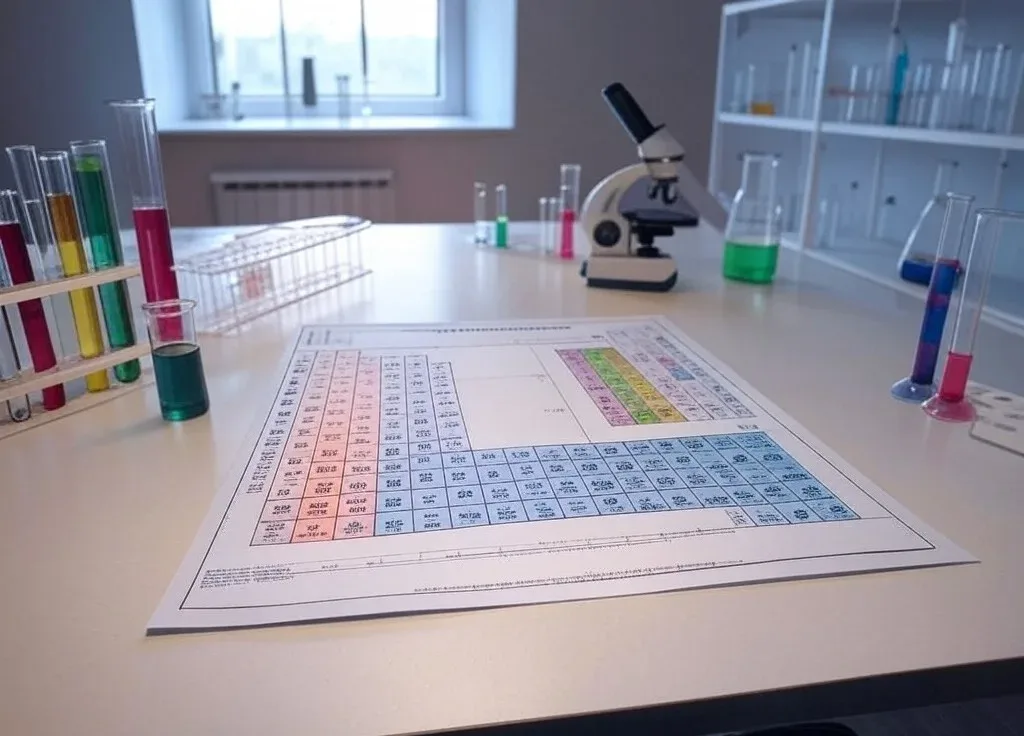
Periodic Table
Table of Contents
- Introduction to Periodic Table Evolution
- The Historical Milestones in Periodic Table Evolution
- Understanding Atomic Structures for Periodic Table Evolution
- Modern Developments and Future of Periodic Table Evolution
- Early Attempts at Classifying Elements
- Mendeleev’s Vision and Its Impact
- The Rise of Atomic Number as a Classification
- Exploring the Significance of Periodicity
- The Expansion Beyond the Known Elements
- The Influence of Quantum Mechanics on Element Organization
- Practical Applications and Industrial Relevance
- Future Perspectives and Unresolved Questions
- References
1. Introduction to Periodic Table Evolution
Chemists have long sought a logical system that arranges elements. Early attempts often relied on complex observations and elemental properties. However, researchers realized that an underlying order existed. As they discovered new elements, they recognized patterns emerging. Today, many consider this organizational tool a cornerstone of chemistry. It guides students, scientists, and industry professionals.
Periodic Table Evolution did not occur instantly. Instead, it involved many incremental steps. Scientists refined ideas, discarded flawed models, and introduced modern concepts. For instance, attempts to classify elements by weight gave way to organizing them by atomic number. Consequently, the modern table provides a stable framework to predict chemical properties and reactivity.
In this introduction, we must acknowledge the challenges scientists faced. They lacked advanced instrumentation and often worked with limited data. Yet, they persevered. Gradually, patterns became clearer, and the concept of periodicity emerged. Thus, the table evolved from a scattered assortment of symbols into a coherent map of matter’s building blocks.
Moreover, the concept extends beyond static representation. Ongoing research uncovers superheavy elements and refines atomic structures. Therefore, this table remains dynamic. It continues to expand as we push the boundaries of known chemistry. Indeed, the history of this system resembles a long narrative of discovery and adaptation. The result is a versatile tool that helps interpret both familiar and unfamiliar chemical behavior.
Today’s generation inherits a robust structure. Periodic Table Evolution continues as scientists probe deeper into the nature of elements, bonds, and molecules.
2. The Historical Milestones in Periodic Table Evolution
Chemistry’s past offers many intriguing attempts to organize elemental knowledge. Early thinkers grouped elements as metals or nonmetals, yet that proved insufficient. By the late 18th century, Antoine Lavoisier’s systematic naming conventions marked initial progress. Eventually, Johann Döbereiner’s triads highlighted patterns. However, these early models lacked a unifying principle. Still, they set the stage for Periodic Table Evolution.
In the mid-19th century, chemists intensified their search for a cohesive framework. John Newlands proposed the Law of Octaves, suggesting every eighth element shared similarities. Although ridiculed, his insight advanced the quest. Soon, Dmitri Mendeleev and Julius Lothar Meyer introduced more refined arrangements. Mendeleev’s table predicted undiscovered elements and their properties with remarkable accuracy. This bold move gained recognition and solidified the notion that elements follow periodic laws.
As discoveries continued, new milestones appeared. The recognition of noble gases demanded an additional column. Henry Moseley’s use of X-ray spectroscopy rearranged elements by atomic number, not atomic mass. Consequently, the puzzle finally aligned. The periodic law gained a solid foundation.
By the 20th century, Glenn Seaborg’s work on transuranic elements expanded the table even further. Thus, each historical milestone contributed layers of understanding. These achievements represent a chain of intellectual breakthroughs. Modern students study a refined structure, but these past efforts remain crucial. Without the cumulative insights of many investigators, Periodic Table Evolution would not have reached its current state of elegance and accuracy.
3. Understanding Atomic Structures for Periodic Table Evolution
At the heart of chemical order lies the atom. Its structure shapes how elements behave. Initially, scientists thought atoms were indivisible spheres. Later discoveries exposed subatomic particles and complex interactions. Understanding these finer details advanced Periodic Table Evolution.
Electrons, protons, and neutrons influence element classification. When researchers learned that atomic number equals proton count, ordering elements became clearer. This realization solved many mysteries. For example, elements once considered out of place found logical homes. Thus, atomic structure dictated periodicity.
Furthermore, electron configurations explain why elements in the same group share similar properties. Sodium, potassium, and rubidium each have a single electron in their outer shell. Consequently, they behave alike. This pattern repeats throughout groups. In addition, scientists recognized that electron shells and orbitals form systematic layers. These patterns shape bonding tendencies, ionization energies, and oxidation states.
Moreover, understanding quantum mechanics refined our view of atomic structure. Complex probability distributions replaced simplistic orbits. This deeper comprehension allowed chemists to predict properties for yet undiscovered elements. As experimental techniques improved, atomic masses, isotopes, and electron affinities became precise. Data accumulated, and the table’s layout reflected these insights.
Today’s periodic table seems stable, yet ongoing research may adjust atomic weight values, discover new isotopes, or identify heavier elements. Indeed, full comprehension of the atom’s nature underpins the table’s logic. The continuous pursuit of atomic-level details assures that as knowledge grows, so does the sophistication of this chemical roadmap.
4. Modern Developments and Future of Periodic Table Evolution
Our current table represents more than a century of collective effort. However, chemists continue to push beyond established boundaries. Laboratories worldwide attempt to synthesize heavier elements at particle accelerators. They add new pieces to the puzzle. These modern efforts shape the future of Periodic Table Evolution.
Moreover, advanced computational models predict properties and stability before synthesis occurs. Consequently, experiments target plausible candidates. Scientists then refine how elements fit within the structure. This iterative process strengthens the table’s predictive power. In addition, advanced spectroscopic techniques measure subtle effects, improving accuracy in atomic weight and electron configuration data.
Researchers also investigate hypothetical “islands of stability,” regions where superheavy elements might exist longer. If found, these discoveries could reshape some boundaries. On another front, technology drives the integration of interactive and digital versions of the table. These innovations enhance learning and research. For example, dynamic visualizations highlight periodic trends and facilitate rapid comparisons.
Beyond discovery, modern chemists embrace interdisciplinary approaches. Material science, medicine, and energy sectors all rely on this structure. By understanding element placement and behavior, researchers design catalysts, drugs, and novel alloys. This synergy drives socio-economic impact while guiding fundamental science.
The periodic table’s future remains bright. Ongoing refinement ensures it will remain a key scientific tool. As knowledge expands, we adapt, update, and improve the framework. Ultimately, Periodic Table Evolution continues, fueled by curiosity, innovation, and a persistent quest to understand the building blocks of matter.
5. Early Attempts at Classifying Elements
Before a coherent structure emerged, scientists struggled with crude grouping methods. Alchemists considered philosophical principles, while some grouped elements by appearance or use. However, these simplistic attempts lacked a unifying principle. Iron, lead, and gold differed in obvious ways, but no deeper rationale guided their order.
Gradually, chemists introduced systemized methods. Lavoisier’s classification of elements into gases, metals, and nonmetals offered a preliminary scaffold. Yet, many elements did not fit neatly. Likewise, Berzelius introduced atomic weight measurements, hoping to find patterns. These efforts hinted at order but did not solve the puzzle.
During this period, progress was incremental. Some proposed alphabetical lists, while others arranged elements by reactivity. Inevitably, confusion arose because no overarching law existed. Nonetheless, each attempt contributed a piece to the eventual framework. As new elements were discovered, inconsistencies grew, urging scientists to find a better method.
The absence of a standardized table limited chemical education and research. Students memorized properties in isolation, and predicting new elements remained guesswork. Although these early attempts seem rudimentary, they set the stage for breakthroughs. Scientists refined measurements, improved techniques, and recorded more accurate data.
Eventually, clearer patterns emerged. The realization that properties repeated periodically allowed researchers to move beyond guesswork. This transition marked the beginning of a structured approach. Later innovators would build upon these foundational efforts, transforming scattered data into a logical, predictive map of chemical behavior.
6. Mendeleev’s Vision and Its Impact
Dmitri Mendeleev’s greatest achievement lay in recognizing a pattern others missed. By arranging elements in order of increasing atomic mass and aligning those with similar properties beneath each other, he created a remarkable prototype of today’s periodic table. Notably, he left gaps where no known elements fit. This bold decision proved crucial.
When gallium and germanium were later discovered, they matched Mendeleev’s predictions closely. Consequently, confidence in his approach soared. His foresight cemented the concept that the table was more than a descriptive tool. It was predictive. This established periodicity as a guiding principle.
Although Mendeleev’s table was not perfect—some elements seemed out of order—his methodology inspired others to refine it. Later, Moseley’s work provided a more fundamental ordering principle: atomic number. Still, Mendeleev’s legacy endures. He propelled the field forward, demonstrating that the elements followed a coherent order.
Table: Selected Elements Predicted by Mendeleev
Predicted Element | Actual Discovery | Predicted Properties vs. Actual |
---|---|---|
Eka-Aluminium (Ga) | 1875 (Gallium) | Density, melting point accurate |
Eka-Silicon (Ge) | 1886 (Germanium) | Density, atomic mass close match |
Mendeleev’s contribution transformed chemistry. Students and professionals embraced the table as a reference. They could now infer missing elements, anticipate properties, and rationalize chemical behavior. His vision clarified relationships and inspired confidence in periodicity. Without his insight, the journey of building a coherent structure might have taken far longer.
7. The Rise of Atomic Number as a Classification
The early tables arranged elements by atomic mass, which led to anomalies. For instance, iodine and tellurium defied that pattern. Although Mendeleev forced these elements into positions based on properties, the reasoning felt incomplete. A better system awaited discovery.
Henry Moseley’s experiments using X-ray spectroscopy provided the missing link. He found that each element’s identity stemmed from its atomic number, the count of protons in its nucleus. Arranging elements by atomic number eliminated irregularities. The periodic law gained a robust and quantifiable foundation.
This shift represented a profound advance. Properties aligned more consistently, and the placement of every element made sense. Differences that once seemed puzzling vanished under this new lens. The periodic table became a harmonious structure rather than a forced alignment.
In addition, the concept of atomic number opened new avenues of research. Scientists measured elements more accurately and predicted new ones with greater assurance. This approach clarified why certain elements shared characteristics. Their similar valence electron configurations stemmed from the systematic increase in proton number.
No longer did chemists rely on approximations or guesswork. They embraced a core principle tied directly to atomic structure. Today, the ordering by atomic number remains fundamental. It unifies theory, practice, and application. Atomic number classification ensured that the periodic table stands as a reliable resource. It guides scientists in exploring matter’s building blocks and interpreting their vast complexity.
8. Exploring the Significance of Periodicity
Periodicity underpins the table’s logic. Elements in the same group often share valence electron configurations. Consequently, they exhibit similar chemical reactivity and bonding tendencies. This pattern allows scientists to predict unknown properties, speeding research and innovation.
For example, alkaline earth metals show gradual changes in hardness and reactivity down the group. Halogens demonstrate consistent patterns in electron affinity. These recognizable trends shape our understanding of how matter behaves. They also guide industrial processes, material design, and pharmaceutical development.
Table: Periodic Trends in Selected Properties
Trend | Across a Period (Left to Right) | Down a Group (Top to Bottom) |
---|---|---|
Atomic Radius | Decreases | Increases |
Ionization Energy | Increases | Decreases |
Electron Affinity | Generally Increases | Generally Decreases |
By analyzing these trends, chemists relate structure to behavior. For instance, reducing agents tend to appear in groups with lower ionization energies. Likewise, elements forming stable anions cluster in regions of higher electron affinity. Patterns emerge, allowing logical reasoning rather than memorization.
This understanding impacts countless fields. Material scientists select elements that yield desired conductivity or durability. Medicinal chemists choose elements and their compounds for targeted activity. Engineers design corrosion-resistant alloys by capitalizing on predictable patterns. The benefits extend beyond pure science.
Ultimately, periodicity transforms the table into a roadmap. It reveals how elemental properties shift in logical ways. This foundation underlies the table’s enduring value.
9. The Expansion Beyond the Known Elements
As the known elements filled the periodic table, scientists pushed beyond natural boundaries. They synthesized heavier and more unstable elements. Each new addition tested the limits of chemistry and nuclear physics. These superheavy elements exist for fractions of a second. Yet, they confirm that the pattern continues.
Their fleeting existence challenges our understanding of stability. However, careful measurements confirm that they fit into predicted slots. Although verifying properties is difficult, these discoveries reinforce the table’s overarching logic.
Expanding the table requires sophisticated equipment and expertise. Particle accelerators produce collisions that fuse atomic nuclei. Researchers then analyze the decay patterns. Even if these elements vanish quickly, they leave clues. Their brief presence validates theoretical models. It also encourages the search for “islands of stability,” regions where superheavy atoms may persist longer.
This ongoing work keeps the table vibrant. Scientists refine theoretical predictions, explore electron configurations, and envision entirely new blocks of elements. As the table expands, chemists must rethink how to represent these new realms. Interactive digital platforms help present the updated structure. They allow users to zoom in on unfamiliar corners of the table and compare properties instantly.
In addition, these discoveries fuel interdisciplinary collaborations. Physicists, chemists, and engineers unite in pursuit of knowledge. Each new element bridges gaps, opening fresh questions. The table is not static; it evolves with our growing capabilities. This continual growth ensures that the table remains a living document of our scientific progress.
10. The Influence of Quantum Mechanics on Element Organization
Quantum mechanics revolutionized our understanding of atomic structure. Traditional models could not explain electron distribution within atoms. Quantum theory introduced orbitals, probability distributions, and quantized energy levels. By embracing these complex ideas, scientists refined predictions about element behavior and arrangement.
This deeper understanding supports Periodic Table Evolution on a theoretical level. Electron configurations determine how elements interact. For instance, transition metals owe their diverse chemistry to partially filled d orbitals. Rare-earth elements, defined by their f orbitals, have subtle differences that affect magnetic and optical properties.
With quantum mechanics as a foundation, chemists interpret trends more accurately. They understand why ionization energies rise across a period or why certain elements adopt unusual oxidation states. Theoretical calculations predict unknown properties, guiding experimentalists toward promising research directions. Thus, quantum theory and experimentation form a feedback loop.
Moreover, quantum mechanics provides a language to describe newly synthesized elements. As heavier nuclei hold more protons and electrons, these interactions become complicated. Without quantum theory, making sense of superheavy elements would be nearly impossible. It offers a framework to anticipate stability, electron arrangement, and reactivity.
This integration of theory and data ensures that the periodic table reflects the most advanced scientific knowledge. Rather than relying on empirical observation alone, today’s chemists employ quantum principles to guide their reasoning. In doing so, they continually refine the structure and expand its predictive capabilities.
11. Practical Applications and Industrial Relevance
The periodic table is not merely an academic tool. Industries depend on it to select and optimize materials. Metallurgists tailor alloys by considering atomic sizes, bonding preferences, and electron configurations. Pharmaceutical companies select elements for medicinal compounds that must balance stability and reactivity. Thus, the table’s predictive power saves time and resources.
Chemical engineers rely on known trends to design catalysts. Understanding how transition metals behave allows them to improve reaction rates and yields. For example, selecting a catalyst from the platinum group might accelerate a crucial step in a drug synthesis. Similarly, battery developers investigate elements that provide better energy density or longer lifespans. Knowledge of periodic trends guides the search for suitable electrode materials.
Environmental engineers consider elemental properties when addressing pollution. They choose elements that capture pollutants or facilitate their decomposition. By harnessing predictive patterns, these professionals tackle global challenges. Moreover, knowledge from Periodic Table Evolution informs waste disposal strategies for radioactive isotopes. Understanding how certain elements decay guides containment plans.
In agriculture, trace elements play vital roles in soil health. Farmers optimize fertilizer blends by considering how plants utilize nutrients. Similarly, semiconductor manufacturers exploit periodic trends in conductivity to develop more efficient chips. Solar cell efficiency benefits from selecting elements with optimal band gaps.
From classroom learning to massive industrial complexes, the table informs countless decisions. Its organized logic translates into practical, real-world advantages that shape modern life.
12. Future Perspectives and Unresolved Questions
Despite remarkable progress, mysteries remain. Some predict that superheavy elements might reveal entirely new chemical behaviors. Others wonder if the concept of periodicity itself might shift at extreme proton numbers. As scientists probe these regions, they face experimental and theoretical challenges.
In addition, researchers consider how changing fundamental constants might affect periodicity. While speculative, these questions encourage deeper exploration. Understanding these frontiers drives further Periodic Table Evolution. Emerging computational methods, machine learning, and artificial intelligence suggest new strategies. They help predict properties faster, design experiments more efficiently, and interpret complex data.
Questions about how best to represent elements persist. Traditional two-dimensional layouts cannot fully capture all nuances. Some propose three-dimensional or spiral representations, emphasizing different relationships. These visualizations may help students grasp abstract concepts more intuitively.
Moreover, interdisciplinary collaborations will likely grow. Physicists studying nuclear stability, astrophysicists considering elemental abundances in stars, and biologists examining trace elements in organisms all benefit from periodic insights. The table can bridge fields, prompting creative solutions to global challenges.
Uncertainty inspires innovation. Each answer raises new inquiries, ensuring that this grand structure continues to evolve. The future lies open, with potential breakthroughs on the horizon. As we uncover more about atomic structure and nuclear forces, we refine our understanding. Ultimately, the periodic table remains a dynamic symbol of human curiosity, knowledge, and the never-ending quest to decode the universe’s fundamental order.
13. References
- International Union of Pure and Applied Chemistry (IUPAC): https://iupac.org
- Royal Society of Chemistry: https://www.rsc.org
- IUPAC Periodic Table of Elements: https://iupac.org/what-we-do/periodic-table-of-elements/
- Nature Chemistry Periodic Table Resources: https://www.nature.com/subjects/periodic-table
- Commission on Isotopic Abundances and Atomic Weights (CIAAW): http://ciaaw.org/