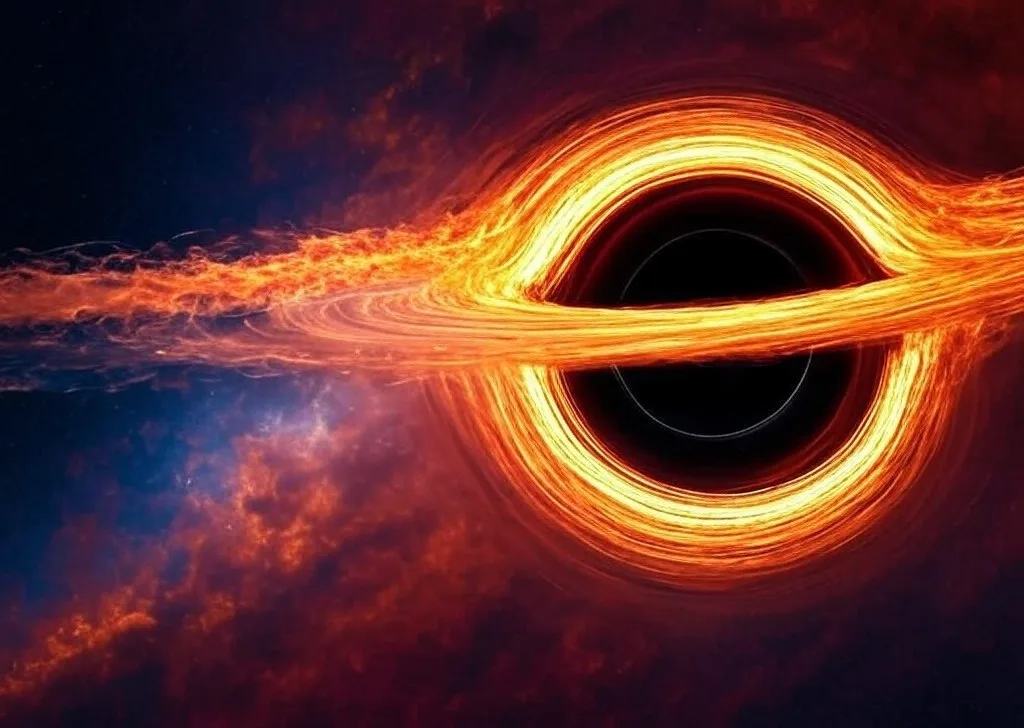
Black Holes
Table of Contents
- Introduction
- The Cosmic Setting: How Black Holes Fit Within the Universe
- Gravitational Collapse and the Formation of Black Holes
- Stellar Evolution Leading to the Formation of Black Holes
- The Role of Density and Pressure in the Formation of Black Holes
- Supermassive Black Holes at Galactic Centers: Exploring Their Formation of Black Holes Origins
- The Event Horizon and the Singularity
- Accretion Disks and Jets
- Observational Evidence: Detecting Black Holes
- Effects on Surrounding Matter and Spacetime
- Primordial Black Holes and Their Mysteries
- Theoretical Models and Simulations
- Future Studies and Research Opportunities
- Conclusion
- References
1. Introduction
The universe extends across unimaginable distances and timescales. Astronomers probe distant galaxies and attempt to understand the darkest corners of existence. Among the universe’s most mysterious entities are black holes. They form when matter compacts into an incredibly small region. Astronomers seek to grasp their origins and nature.
Black holes represent one of the most extreme gravitational phenomena. Researchers study them to learn about the laws of physics under intense conditions. Scientists believe the Formation of Black Holes occurs when gravitational collapse dominates over all opposing forces. Consequently, matter falls inward, leaving behind no stable structure.
In fact, black holes challenge our understanding of space and time. Their interiors remain hidden behind event horizons from which nothing escapes. Moreover, each black hole holds clues to the earliest moments of cosmic history. Although invisible, their influence on surrounding matter announces their presence. By studying them, experts uncover details of stellar evolution and galaxy formation.
This article explores how these objects emerge. It examines the life cycles of massive stars and the extreme conditions that lead to the Formation of Black Holes It also discusses supermassive black holes at galactic centers and considers theoretical models. Furthermore, it looks ahead to what future research might reveal. By the end, readers will appreciate the complexity and grandeur involved in forging these cosmic titans.
2. The Cosmic Setting: How Black Holes Fit Within the Universe
Our universe contains countless galaxies, each hosting billions of stars. Within this grand tapestry, black holes occupy a special role. They lie at the end of certain stellar lifecycles. Additionally, they seem central to the growth of galaxies. Many galaxies, including our own Milky Way, host supermassive black holes at their centers. Yet how do these entities emerge?
Within stellar environments, pressure from nuclear fusion opposes gravitational collapse. Eventually, stars use up their fusion fuel. Without sustained outward pressure, the core succumbs to gravity. In some cases, this process leads to the Formation of Black Holes. Not all stars produce them. Only those with enough mass reach the required conditions.
Understanding their place in the cosmic scheme remains important. Black holes regulate star formation in their host galaxies. Their accretion disks emit powerful radiation that shapes the interstellar medium. Moreover, jets emerging from their poles can influence large-scale structures. Thus, these objects drive galactic evolution across cosmic timescales.
Scientists look to gravitational waves and electromagnetic signals to confirm their influence. New data from modern observatories reveals intricate details. Consequently, the existence of black holes is no longer theoretical. Instead, it stands as confirmed reality. As we uncover more about the Formation of Black Holes, we better understand their cosmic function. Their presence enriches our comprehension of how galaxies, stars, and cosmic matter interact and evolve.
3. Gravitational Collapse and the Formation of Black Holes
Gravity shapes the universe, pulling matter together into stars, planets, and galaxies. Yet gravity can also compress matter beyond normal states. When a star’s core collapses, it may surpass a critical threshold. This threshold results in the Formation of Black Holes, transforming dying stars into regions of spacetime with intense gravity.
As a massive star exhausts its nuclear fuel, fusion slows down. The outward radiation pressure decreases, allowing the core to contract. When the mass is high enough, no known force can stop the collapse. Consequently, densities increase until even light cannot escape.
This collapse happens rapidly. Within seconds, the star’s core shrinks to an incredibly small volume. Outside layers may explode in a supernova blast. The remnant core becomes a new black hole. Although invisible, it interacts strongly with nearby matter. Observers infer its presence through gravitational effects and emitted radiation from hot accretion disks.
This process remains a fundamental piece of astrophysics. Scientists use numerical simulations to understand it. By analyzing the Formation of Black Holes through computer models, researchers refine their theories. With ongoing improvements in computational power, these models grow increasingly realistic. They reveal not only how black holes form, but also their likely masses, spins, and environments.
4. Stellar Evolution Leading to the Formation of Black Holes
Stars begin life in collapsing gas clouds. Over millions of years, they ignite fusion at their cores. Their fate depends on mass. Massive stars live shorter, more violent lives. Eventually, their cores collapse, causing the Formation of Black Holes.
Table 1: Stellar Mass vs. Final Remnant
Initial Star Mass (Solar Masses) | Final Remnant |
---|---|
< 8 | White Dwarf |
8 – 20 | Neutron Star |
> 20 | Black Hole (via Formation of Black Holes) |
These thresholds are approximate. Many factors influence a star’s evolution. Metallicities and rotational speeds adjust outcomes. In general, very massive stars burn through fuel quickly. When fusion ends, the core collapses under immense gravity. No internal mechanism halts this collapse, resulting in a black hole.
Observations of supernova remnants link to black hole births. Astronomers detect intense X-ray sources where massive stars once shone. Moreover, binary systems containing compact objects confirm their existence. Understanding these relationships helps researchers predict the Formation of Black Holes across cosmic epochs. Consequently, star population studies in galaxies map out their birth rates, masses, and end states.
5. The Role of Density and Pressure in the Formation of Black Holes
During stellar collapse, density and pressure conditions determine outcomes. As fusion wanes, the core contracts. The gravitational force grows stronger. Eventually, conditions approach extremes that lead to the Formation of Black Holes. Here, matter reaches densities beyond typical nuclear matter.
At these scales, quantum mechanics and relativistic effects influence the material. Electrons merge with protons, forming neutrons. In less massive cores, neutron degeneracy pressure stops further collapse, forming neutron stars. Yet in more massive cases, even neutron pressure fails. Nothing counters gravity’s pull. The core continues to shrink, crossing the Schwarzschild radius, creating a black hole.
This final step defines the most compact object known. Gravity curves spacetime so strongly that escape becomes impossible. The event horizon marks the point of no return. Inside, densities approach the unimaginable. Conditions test known physics and may reveal new insights about matter.
Astrophysicists simulate this process with complex equations. They incorporate nuclear physics, equations of state, and relativistic gravity. These tools help clarify how different initial conditions influence the final remnant. By refining these models, scientists improve predictions about the Formation of Black Holes. This knowledge also helps identify signatures in gravitational waves, linking theory to observations.
6. Supermassive Black Holes at Galactic Centers: Exploring Their Formation of Black Holes Origins
Galactic centers often host supermassive black holes. These giants can weigh millions or billions of solar masses. Their presence raises key questions. How did such enormous objects form so early? Understanding their Formation of Black Holes at colossal scales demands special explanations.
Some theories propose direct collapse of massive gas clouds. Others suggest smaller seed black holes merge and grow over time. Moreover, the rapid growth of early quasars hints at efficient accretion. Accretion disks feed these black holes, allowing them to gain mass quickly. Interactions with surrounding matter accelerate their expansion.
Observations from the Hubble Space Telescope and ground-based observatories confirm their ubiquity. Bright quasars at high redshifts indicate that supermassive black holes formed within the first billion years. Computational models test scenarios involving dense gas reservoirs. Researchers use these models to determine if they can reproduce the observed population.
The environments of galactic cores differ from stellar collapse sites. Gas dynamics, galaxy mergers, and dark matter halos contribute. By linking theory, simulation, and observation, scientists hope to reveal the exact path. Thus, understanding the Formation of Black Holes at this scale offers a window into the cosmos during its earliest eras.
7. The Event Horizon and the Singularity
A black hole’s defining feature is its event horizon. This boundary separates the interior from the rest of the universe. Within it, escape is impossible. Matter crosses the horizon and continues inward. Eventually, it reaches a central region of infinite density and zero volume: the singularity.
The event horizon size relates to the black hole’s mass. Larger masses produce bigger horizons. In principle, an external observer never sees matter cross the horizon. Time dilation makes it appear to slow and fade. Inside, though, the descent continues.
Scientists still strive to understand singularities. They reflect conditions where current theories fail. Quantum gravity concepts might resolve these mysteries. The singularity’s formation accompanies the end stages of stellar collapse and the Formation of Black Holes. Future discoveries in quantum gravity could alter our understanding of these extreme interiors.
Moreover, the horizon itself can radiate. Hawking radiation, a quantum effect, might cause black holes to slowly evaporate over enormous timescales. Although this process is gradual, it hints that black holes are not eternal prisons. Rather, they fit into cosmic evolution in subtle ways.
8. Accretion Disks and Jets
Black holes do not always exist in isolation. They often interact with nearby matter. Gas and dust can spiral inward, forming accretion disks. These disks heat up and emit intense radiation, making black holes visible indirectly. Accretion plays a role in black hole growth and can influence the environment.
Jets of charged particles can also emerge from the vicinity of black holes. These jets blast out into space at nearly light speed. They shape the intergalactic medium and can suppress star formation in galaxy clusters. Understanding accretion and jet formation helps explain how black holes gain mass after their initial Formation of Black Holes.
Observations with radio telescopes and X-ray observatories provide key data. Astronomers detect relativistic jets extending thousands of light-years. Such dramatic phenomena illuminate black holes’ energetic nature. Furthermore, the study of jets reveals how angular momentum, magnetic fields, and plasma physics interact near extreme gravity.
These processes highlight black holes as active participants in cosmic ecosystems. They regulate surrounding matter, radiation, and even magnetic fields. By examining accretion disks and jets, astronomers gain insight into the balance between feeding black holes and shaping galaxies over eons.
9. Observational Evidence: Detecting Black Holes
Detecting black holes poses challenges since they emit no light. However, scientists rely on indirect signs. Accretion disks, gravitational lensing, and orbital motions of nearby objects reveal hidden black holes. The Laser Interferometer Gravitational-Wave Observatory (LIGO) has also confirmed mergers of black holes through gravitational waves.
Table 2: Key Observational Methods
Method | Evidence Provided |
---|---|
Accretion Disk Emission | High-energy radiation |
Stellar Orbital Motion | Mass estimates via dynamics |
Gravitational Waves | Merger events, mass, and spin |
Each method provides complementary clues. When massive stars collapse, the Formation of Black Holes leaves behind a compact object. Astronomers detect related X-ray binaries. In such systems, matter from a companion star falls into the black hole’s potential well. The resulting emission pattern indicates a hidden, compact object.
Meanwhile, observing stars orbiting the Galactic Center’s black hole provides direct mass estimates. These orbits confirm an invisible, supermassive object. Recent images of M87’s black hole shadow from the Event Horizon Telescope deliver visual confirmation. Thus, detection techniques continue to improve, providing robust support for black hole theories.
10. Effects on Surrounding Matter and Spacetime
Black holes exert profound gravitational influence. Their intense gravity distorts spacetime around them. Nearby objects experience strong tidal forces. Gas and dust spiral inward, heating up. This energy release can trigger cosmic fireworks. In galaxy clusters, jets from supermassive black holes affect gas distribution, quenching star formation.
Over cosmic times, black holes shape large-scale structures. Their outflows can redistribute matter, heating the intracluster medium. Consequently, star formation rates shift, influencing the evolutionary paths of galaxies. Although they result from stellar collapse and the Formation of Black Holes, they evolve into key cosmic architects.
Gravitational lensing by black holes bends light from background sources. This phenomenon allows astronomers to study distant galaxies. Moreover, black holes can influence gravitational wave signals from inspiraling objects. Such signals offer insights into mass, spin, and merger histories.
Understanding these effects refines our view of cosmic evolution. By integrating black holes into models, scientists predict how galaxies change over billions of years. Ongoing observations and simulations strive to capture these subtle dynamics. Thus, black holes link small-scale physics to large-scale cosmic structures.
11. Primordial Black Holes and Their Mysteries
Not all black holes form from stars. Some might have originated in the early universe. These primordial black holes, if they exist, could date back to the first fractions of a second after the Big Bang. Theories suggest density fluctuations in the young cosmos led to clumps collapsing into black holes. This early Formation of Black Holes predates stars.
Primordial black holes range from tiny to enormous. Those of smaller mass may have evaporated via Hawking radiation. Larger ones might persist. They could serve as a dark matter candidate, though current constraints limit their possible abundance. If discovered, they would open a new window into the very birth of our universe.
Detecting primordial black holes is challenging. Astronomers search for lensing effects on distant stars. They also seek gravitational wave events involving unusually low-mass black holes. Any confirmed detection would illuminate conditions in the infant cosmos. It would show how gravity interacted with matter before stars and galaxies formed.
Continued observations from next-generation detectors may reveal their secrets. If primordial black holes exist, they bridge cosmology and quantum gravity. They highlight how extreme conditions shaped matter into these bizarre objects long before stellar collapse took place.
12. Theoretical Models and Simulations
Theoretical models underlie our understanding of black hole formation. General Relativity provides the framework for describing gravitational collapse. However, at extreme densities, new physics may arise. Quantum gravity theories attempt to unify gravity with quantum mechanics. Such efforts seek to explain singularities and the nature of spacetime inside black holes.
Simulations advance this field. Powerful supercomputers run complex codes that solve Einstein’s equations. They track matter from a collapsing star’s core through the Formation of Black Holes to the final stable configuration. Models include magnetic fields, rotation, and turbulence. By adjusting parameters, researchers explore a range of scenarios.
Simulations also inform gravitational wave research. When two black holes merge, they emit gravitational waves. By comparing observed wave patterns to simulated predictions, scientists infer properties of the merging objects. This approach refines both theory and observation.
In addition, simulations help predict electromagnetic counterparts. They reveal how accretion flows evolve and how jets form. These predictions guide astronomers searching for multi-messenger signals. Over time, better models and more realistic simulations bring us closer to a full understanding. They confirm or challenge theoretical assumptions, ensuring our knowledge remains tested by data.
13. Future Studies and Research Opportunities
Black hole research stands at a dynamic frontier. Upcoming observatories will extend our reach. Next-generation gravitational wave detectors, such as LISA, will probe merging black holes at earlier cosmic times. Proposed X-ray missions may capture better views of accretion disks.
Space-based telescopes could map black holes across the universe. By combining gravitational wave data with electromagnetic signals, we form a richer picture. This multi-messenger approach advances our comprehension of the Formation of Black Holes and their evolving roles. It also uncovers details about cosmic history, star formation rates, and the distribution of dark matter.
Additionally, theoretical breakthroughs are on the horizon. Advances in quantum gravity, string theory, and holographic principles may clarify singularities. Interdisciplinary collaborations will merge astrophysics, cosmology, and particle physics. Such synergy can produce fresh insights into these enigmatic objects.
Education and public engagement also remain important. As scientists uncover more facts, they share findings with broader audiences. This ensures that the cosmic drama of black hole birth and evolution captivates minds worldwide. The journey to understand them continues, promising many surprises ahead.
14. Conclusion
Black holes stand among the universe’s greatest enigmas. From stellar collapse to the largest structures at galactic cores, they play an essential part in cosmic evolution. The Formation of Black Holes arises when gravity overpowers all resistance, compressing matter into regions of extreme density. Studying their origin reveals much about physics, cosmology, and the fate of massive stars.
Although once theoretical curiosities, black holes are now established cosmic actors. Observations confirm their existence. Gravitational waves and electromagnetic signals detail their properties. Supermassive black holes anchor galaxies, influencing star formation and gas distribution. Primordial black holes, if confirmed, would rewrite early universe history.
As technology advances, we will learn more. Our simulations grow more sophisticated. Our detectors become more sensitive. Each discovery raises new questions, driving further exploration. The quest to comprehend black holes reflects humanity’s broader desire to understand the cosmos. It shows that by probing the darkest corners of space-time, we illuminate the deepest truths of reality.
15. References
- NASA: https://www.nasa.gov/black-holes
- European Southern Observatory (ESO): https://www.eso.org/public/news/eso2409/
- Event Horizon Telescope Collaboration: https://eventhorizontelescope.org
- LIGO Scientific Collaboration: https://www.ligo.org
- Einstein Online (Max Planck Institute): https://www.einstein-online.info/en/spotlight/black-holes/
“Great content, learned a lot from this post!”